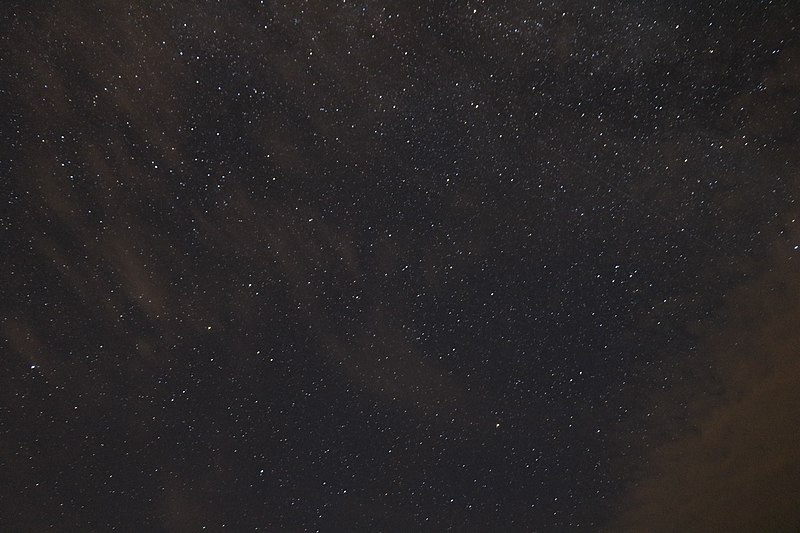
In my last post I tried to give an introduction to the subject of space habitats in as neutral a fashion as possible. I am sure many of you readers were asking yourself “What is Anders’ personal opinion about the best design for a space habitat?”.
I am actually very happy that you were asking yourself this. Because I have spent the last 25 years thinking about this very question. This is about as good as it gets since I have neither experience, nor education when it comes to astronomy, space travel, large-scale construction engineering or anything else relevant for space habitats. In short, I am an amateur. On the other hand, when it comes to space habitats, so is almost everyone else too.
Lighten up
These days most prospective space habitat designers seem to be going for some sort of cylinder design not unlike Kalpana One that I reviewed in my last post. The basis is a stable (meaning wide and low) cylinder with multiple interior levels.
This thing with the levels is very important. And very clever. By stacking multiple space habitats on top of each other one can dramatically lower the amount of radiation shielding necessary to cover the entire space habitat. In fact, it is so clever one can be excused for asking why no one thought about this in the 1970s, when most space habitat designs were explored?
The reason for this is simple. Stacking multiple space habitats on top of each other makes it impossible or at least very difficult to light up the habitat using solar light. The thing that has changed since the 1970s is LED lighting. Today it is perfectly possible to make a habitat with only artificial lights and thereby with a much greater flexibility in how the habitable zones are arranged.
LED lights are great for many things but personally I am not convinced they make for great space habitats. Not primarily because artificial light is any worse than natural light. I do believe that, but space habitats are not very natural in the first place, and artificial light will probably not make a whole lot of difference there. The real drawback in my opinion is the cost. Not in energy terms, because space is brimming with easily accessible solar power. But rather the maintenance cost.
According to the Kalpana One project their 3,000 inhabitants will require 150,000 square meters of agricultural land. To illuminate this area will require 150 MW of electrical power. Assuming a single LED light provides 10W, this amount of artificial light would require 15 million LED lights.
A decent LED light will have a lifespan of maybe 50,000 hours. If the lights break down at an even rate that means that the maintenance personnel at Kalpana One will have to replace 300 LED lights per hour, every hour, all year round. Half a dozen or so of Kalpana One’s inhabitants will have to work full-time replacing broken lights.
And then there is the question of where the LED lights should come from. They can either be imported from Earth at an eye-watering cost. Or they could be produced in space which means that a quite complicated production line needs to be set up only to produce light, the one thing that is in abundance in space. To me it makes no sense to go through all this trouble when some mirrors can solve the problem just as easily.
Shape up
Having decided that a viable space habitat needs natural light should force us back to the designs of the 1970s. Or not. I am determined that there must be a way to combine stacked levels of space habitats with natural solar light. The rest of this article rests heavily on the assumption that this is possible.
But first, what shape should a space habitat have? The foremost consideration when choosing a shape is to maximize internal volume while minimizing the outer area and thus the required radiation shielding.
Kalpana One and its derivatives opt for the cylinder shape. This is not wrong since a cylinder gives plenty of volume for a limited amount of radiation shielding and, in contrast to the sphere shape, this volume is non-sloping which makes it easy to stack levels on top of each other.
There is one obvious drawback with the cylinder shape that I can think of: Namely that it shields a lot of unwanted volume. A space habitat really has very little use for the zero gravity volume close to the axis of rotation. If the cylinder in question is long it will still be cheaper to cover the caps with shielding than it is to cover the roof above the habitable areas. But for a short and flat cylinder the caps will require more resources than the roof.
The difference between long and short cylinders is shown in the image below (trigger warning: this article contains numerous lousy artworks produced with Microsoft Paint). The image shows cross-sections of cylindrical space habitats. The red in the image denotes radiation shielding while black is non-shielded structural elements. As you can see the short and flat cylinder (the one on the right) requires much more shielding material per area of livable area compared to the longer and more compact cylinder on the left:
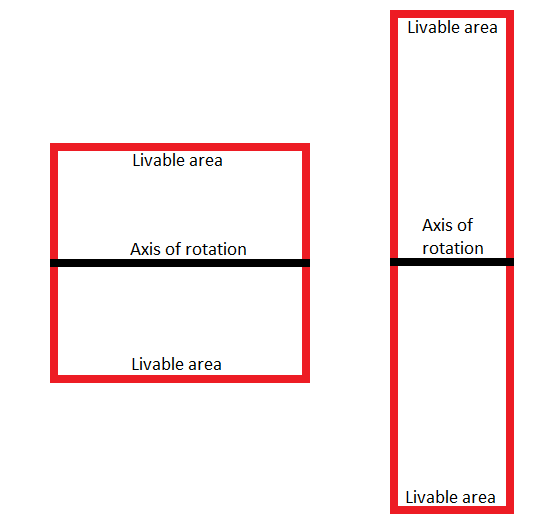
This problem can be mitigated by covering the roof of the habitable zone instead of the entire cylinder. Like this:
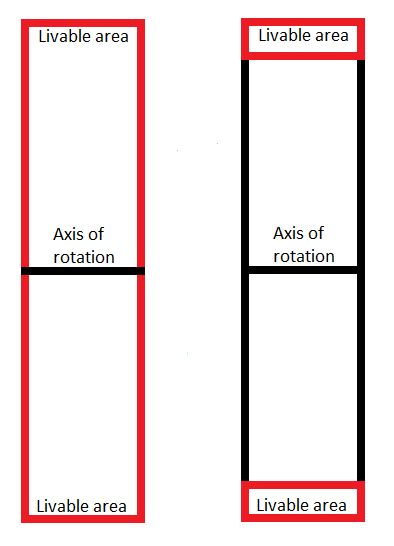
In practice, the cylinder has been transformed into a wheel or a torus, making it all very similar to the Stanford torus. The problem with the Stanford torus is that light is supposed to come in from the top, making it impossible to stack multiple levels of livable area on top of each other.
This should be solvable, though. With some nifty use of mirrors it should be possible to take in light from the side while still dispersing it from above in the actual habitat. I have tried to illustrate the point below (although my ability to illustrate concave and convex mirrors is clearly very limited):
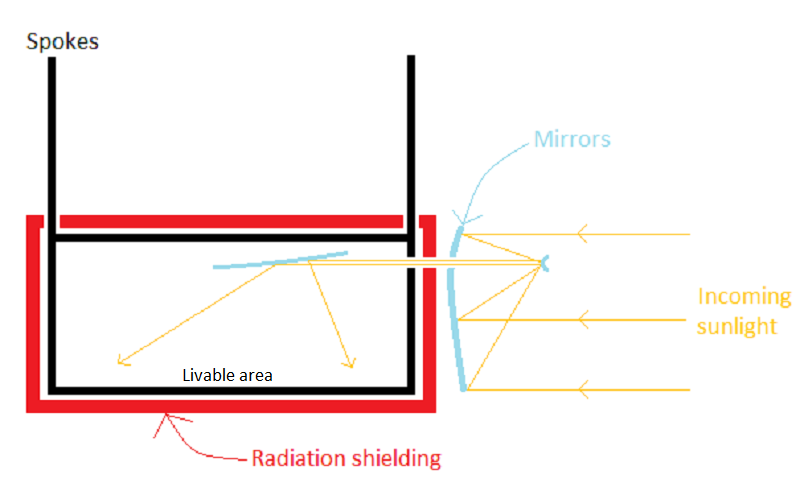
The use of a small shaft to bring light into the interior of the habitat has a clear advantage in that it limits the entry point for harmful radiation. Getting light in while keeping radiation out is otherwise usually done with a chevron design, both the Stanford torus and the original Kalpana One intended to use this. With chevrons, light is shuttled between mirrors that reflect the light while absorbing cosmic radiation, like in the image below:
Chevrons work, but increase the total mass of the radiation shield. A light shaft, by contrast, subtracts from the total weight. One problem with a light shaft is that if it is small enough, and the light going through it concentrated enough, it will probably absorb enough heat for the glass in it to overheat, requiring active cooling. This would lead to a host of other problems which are better avoided. There should be some point where the light shaft is large enough to not require active cooling while at the same time small enough to keep out most of the cosmic radiation. However, I have not done any calculations on where this sweet spot is, I just assume that it is somewhere (if problematic, it is always possible to make the shaft longer, that way increasing radiation protection, although this will add weight to the construction).
Stacking it
With light coming in from the side there is nothing preventing the stacking of multiple levels of space habitats on top of each other, like this:
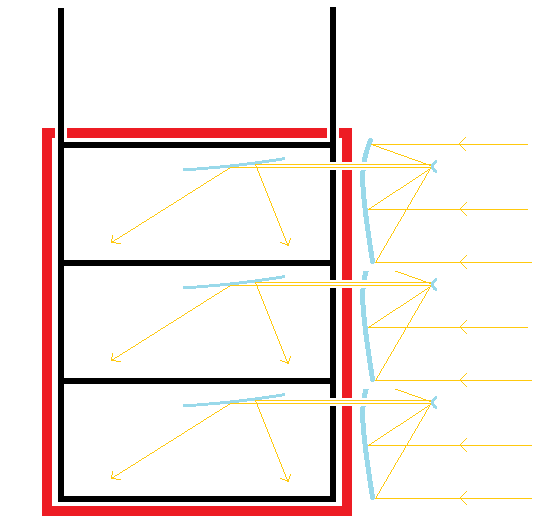
The number of levels one can stack in this manner is somewhat flexible but dependent on the total radius of the habitat. Since the radius (the distance to the axis of rotation) is different for each level, the gravity will also be different for each level. With many levels there might be unacceptable gravity differences between lower and upper levels. However, this is also a reason to make the radius of the habitat as large as possible. Not only will a greater radius decrease the Coriolis forces, it will also decrease the gravity difference between different levels.
Economies of scale are very real when talking about space habitats. For a very small initial space habitat the economy will be different. The radius can hardly be more than a couple of hundred meters. Not primarily because the spokes would be too long and too costly, but mostly due to a large radius giving a large circumference and thus a large, and costly, space habitat. With a radius of 200 meters you can probably only stack a few 20 meter high levels on top of each other. The gravity difference between 240 meter radius and 200 meter radius will be significant.
There are some ways to mitigate this problem, even in a small habitat. One obvious way is to dedicate all the upper levels to agriculture. All habitats require that a majority of the liveable area be dedicated to agriculture. Plants are not as fastidious with gravity as humans, theories are that selectively bred agricultural plants can grow better in a low-gravity environment where less resources are needed for stems and other structural components of plants. Agricultural plants have another advantage: They are not that sensitive to radiation either. In practice this means that if the top levels of a torus-shaped habitat are dedicated solely to agriculture it is probably possible to skip the radiation shielding on top of the habitat. This is very convenient since the spokes of the torus are placed here and would have required the radiation shield to move with the habitat’s rotation inducing artificial gravity and significantly increasing the structural requirements of the construction. The result would look something like this:
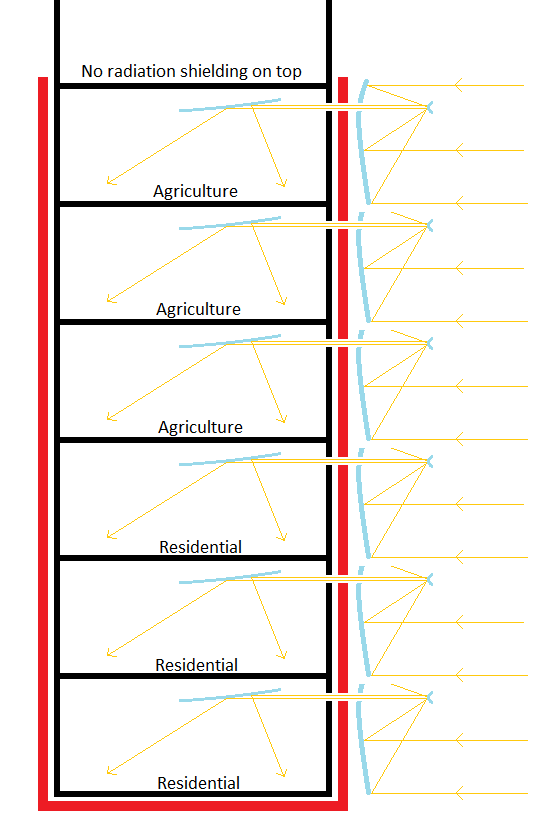
Let there be light (but not too much)
Another problem that is easily visible from these sketches is that the width of the habitable area is dependent on the height, since the amount of collected sunlight cannot be more than the height of each level.
If we want full sunshine on every inch of the habitable area the level width can not be more than the level height. Luckily, we do not need full sunshine in the habitable area. Far from it. Direct sunlight at Earth's distance from the sun is approximately 140,000 lux. This is an incredible amount of light. Down on Earth the light level at noon by the equator is something like 100,000 lux (the atmosphere blocks some of the light). But the sun at noon at the equator is smothering. We do not need that. Rather, we should aim for the sunlight of southern Scandinavia in the early afternoon a day in August, a very pleasant place to be. There direct sunlight is something like 20,000 lux. Even this is probably a bit high for a space habitat considering that sunlight will be constant throughout the day rather than lower in the morning and evening. The bottomline is that a light intensity of 10,000 to 20,000 lux should be enough to make both plants and humans happy.
Only using a tenth of the available sunlight means that each level of the space habitat can have ten times the width than the height. A habitat level that is 20 meters high could thus be 200 meters wide. In practice, it is structurally more challenging to increase the width than the height. And since height is also needed for accommodating all human activities the width/height ratio is unlikely to be a problem.
Side by side
In order to use the expensive radiation shielding optimally one should not only stack habitable areas on top of each other but also on the side of each other. This will take advantage of the fact that a colony only needs sunlight for half of the time, while one half of the colony have night the other half can have day and vice versa. It would look like in the image below:
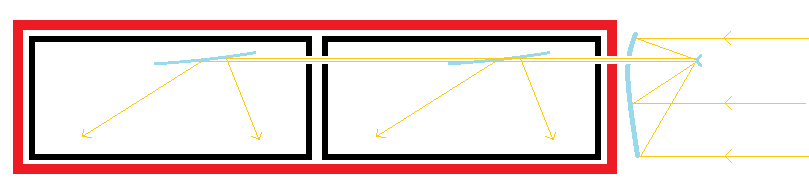
This will require some nifty mirrors (at least in the right half of the colony above) that can either reflect light down to its own colony or let light through to the adjacent colony. This can be achieved using reversible mirrors or some sort of high-tech mirrors that can be ordered to either reflect or let through light in different proportions. I believe this type of material exists although I am not aware of its name or other properties. If it does exist it would also enable something looking like dusk and dawn, which is a nice feature to have.
This set-up also provides a nice solution to the problem of rotational momentum, in a space habitat you ideally want all rotating mass to be net neutral. This is easy to achieve with two similarly sized toruses: Just let them rotate in opposite directions. This way the rotational momentum of the two wheels will cancel each other out making the whole habitat rotationally neutral and thus easier to maneuver.
Connecting the dots
Having two habitat wheels rotating in opposite directions is a nifty way to get rid of most of the rotational momentum. But only most of it. There will always be imbalances due to uneven loads in the habitats. One way to mitigate this is through adjustable weights on the spokes of the wheel. When they move up or down their weight will change due to changed centrifugal force. This can be used to continuously stabilize the rotation of the habitat.
Another way to stabilize the habitat wheel is to connect it to other habitat wheels. In circumstances like these, quantity really has a quality all of its own. The more wheels that are connected to each other the more their rotations and imbalances will cancel each other out and the more stable the whole system of habitats will be.
This is not very hard to achieve, at least not compared to building multiple rotating space habitats. All you need is some sort of bearings on each rotating wheel and a system of scaffolding connecting the habitats. Since the habitats can not be allowed to shade each other they must be located in the same plane (as seen from the sun). This makes distances quite large since even smallish habitats will have to be several kilometers apart. But constructing even very large systems of scaffolding is not that difficult in a zero gravity environment. The result should be something like in the image below (scaffolding in blue):
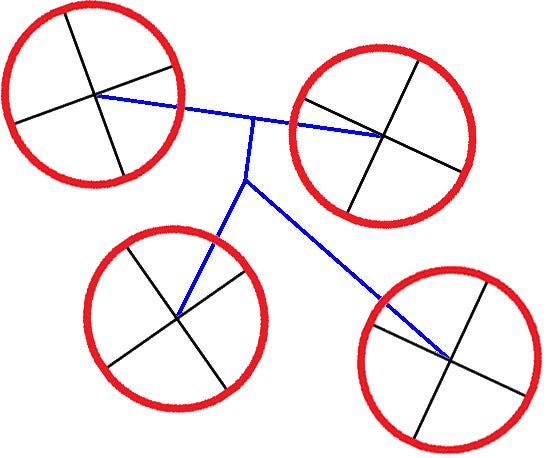
Stabilization is only the first objective. When the scaffolding is already in place it can be used for numerous other things as well. The scaffolding can be used to attach activities that are best done in non-rotating zero gravity to the habitats; things like solar power plants, spaceports and heavy industries. But most obviously the scaffolding can be used for communication and transportation between different habitats.
The scaffolding connecting the habitats will be in the vacuum of space and in zero gravity. This is very similar to the environment proposed by Elon Musk for his hyperloop. The hyperloop is a train supposed to travel at high speeds in vacuum tunnels. Magnetic levitation creates an artificial zero gravity while magnetic propulsion pushes the train forward.
On Earth a hyperloop is difficult and very expensive to make since both vacuum and magnetic levitation are hard to achieve. But in space vacuum and zero gravity are free. Using magnetic propulsion to send pressurized pods between different habitats is both simple and cheap.
Due to the vacuum there are no real speed limits in a system like this. The limiting factor is how much acceleration human passengers can sustain. The main constraint is likely not the traveling time between the hubs of different habitats but rather the time it takes to travel up from the rim of the habitat wheel to the hub in the departing habitat and conversely to travel down from the hub to the rim at the arrival habitat.
A transportation system like this might not seem revolutionary, but it actually is. The speed and low price make transportation possible on a scale that is just not achievable on Earth. The trick lies in constant acceleration rather than constant speed. At constant speed, travel time increases proportionally with distance. This is not the case with constant acceleration. At 1G acceleration (and deceleration) it takes 40 seconds to travel between two habitats 2 kilometers apart. It takes 2 minutes to travel between habitats 40 kilometers apart and 6.5 minutes to travel between habitats 400 kilometers apart.
This abolition of distance means that it will be possible to connect incredible amounts of people with each other. In a densely populated megacity on Earth millions of people can live within 30 minutes travel time of each other. In a megacolony of space habitats billions of people will be able to live within 30 minutes travel time of each other.
Brave new world
Most prospective space colonizers have focused on making space habitats as Earth-like as possible. This was the main objective for Gerard K. O'Neill. His O'Neill cylinders really are very Earth-like. They are probably as Earth-like as it is possible to be for a metal cylinder thrown out in space.
The wheel shaped space habitats I am proposing are not Earth-like in the same sense. The limited volume and low ceiling height guarantee they will never be mistaken for a planet surface. If anything they will probably resemble gigantic greenhouses. That is not Earth but it should be alright. Greenhouses are nice places too.
Instead of emulating Earth I believe it is best to let space be space. Earth will always be superior when it comes to Earth-likeness. But space colonies have some aces up their sleeves nonetheless. The comparison to greenhouses is apt. In a space habitat, just as in a greenhouse, climate is tightly regulated. In practice, this means space habitats will always have perfect weather, no matter what type of weather you consider perfect.
But the real killer app of space is the spatial possibilities that opens up by having three dimensions to work with. And the physical possibilities of not having gravity and atmosphere to contend with. Down on Earth there are very real limitations to the maximum size of societies. These limitations do not apply in space. Using interconnected space habitats it is possible to build societies several magnitudes larger than on Earth. If the tendency for ever more humans to cluster into ever larger agglomerations is to continue there really only is one way forward. That way is upwards.
Thank you, Anders, I am enjoying this series.
I would like to suggest a somewhat different approach. Photosynthesis is a rather inefficient process. It is my understanding that the energetic efficiency is probably only a few percent - although I will be happy to be corrected in this.
Photo cells for space use probably have an efficiency of ~20 -> 30% and have the issue of radiation degradation. Using LED's to make light for plants is going to reduce the effective efficiency - I will assume that the LED's are ~ 30% efficient, for a net efficency < 10%. The concentrating illumination approach will have probably at least 3 mirrors and 2 windows to deal with as well as occulation by various structural elements. I will simply assume 10% losses per interaction, for a throughput of over 55 %.
But hydrolysis has a reasonable efficiency of over 95%. If you do genetic modification of the plants to consume H2 rather than sunlight, you are likely to get a far higher output efficiency than with photosynthesis. They won't formally be plants, but do you care?
I suspect that LED failure has a significant thermal activation component. If you drive them at lower currents they should live much longer. You don't really care that much about the mass of the LED, it is small, and the photonic efficiency should be a bit higher at the lower drive.